Researchers use CRISPR to eliminate HIV in mice, DNA and all
CRISPR is the latest tool adapted from the microbial world to treat infections
Image by Tibor Janosi Mozes from Pixabay
When two species, be they large seabirds and their parasites or tiny bacteria and their viral enemies, share an environment over evolutionary time, they are said to co-evolve. This simply means that they have learned how to deal with each other. Sometimes different species learn to peacefully coexist (and even help each other), but less friendly interactions are also common. This kind of feuding leads to the constant development by each species of new strategies for taking advantage of its enemies, or for defending itself. Recently, researchers have adapted some of these ancient biological tricks, such as CRISPR, to be wielded against the diseases that currently plague us.
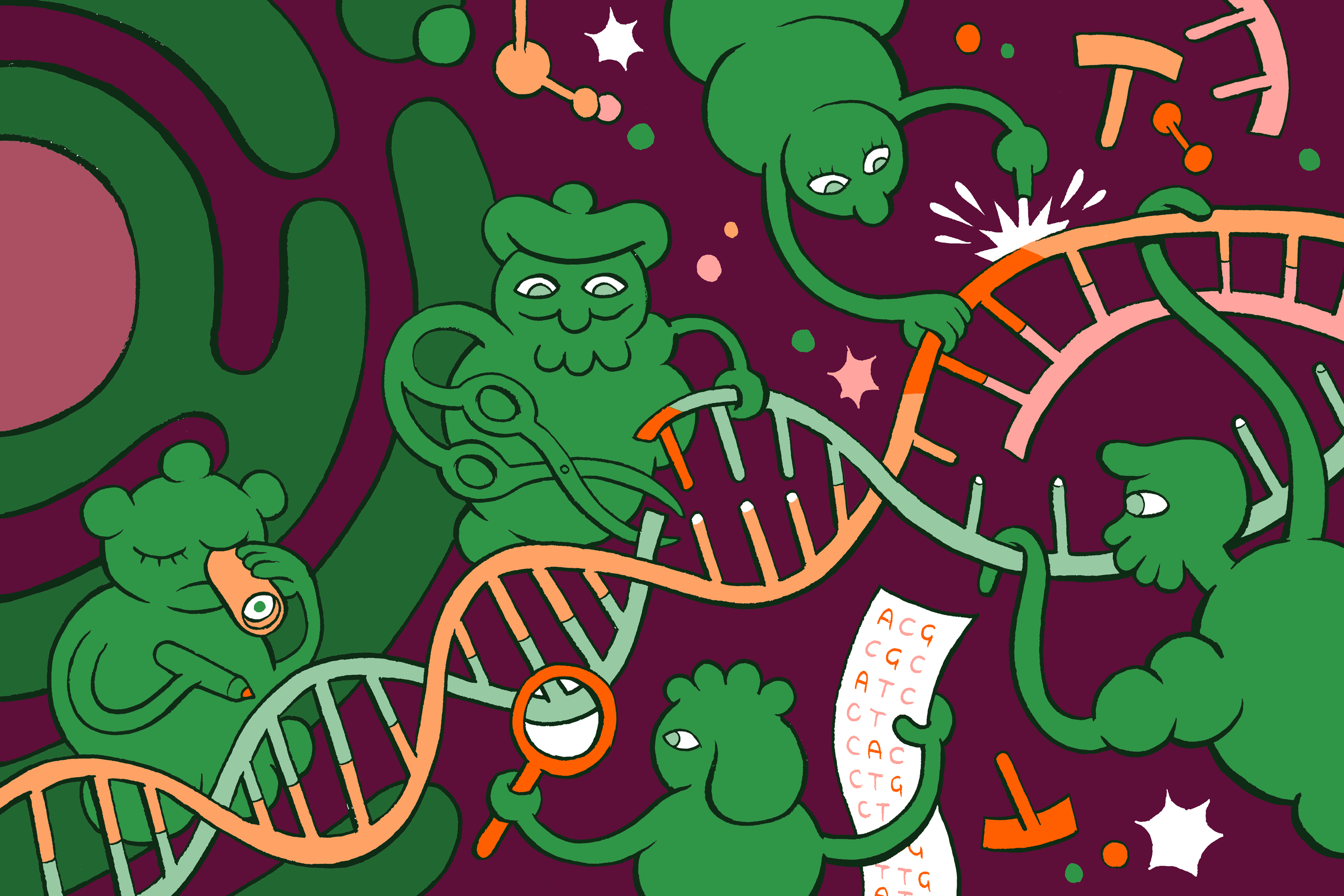
Matteo Farinella
A powerful genome editing technology, CRISPR allows researchers to alter DNA sequences, and has become a versatile tool of modern molecular biology and medicine. But long before humans developed this technology, CRISPR systems naturally evolved in bacteria as antiviral immune responses. It seems fitting, therefore, that some of the most promising applications for CRISPR are developing antiviral therapies.
Recently, American researchers successfully used CRISPR to eliminate HIV in mice. HIV is a retrovirus — a type of virus that inserts copies of its own genetic code into the genomes of the cells that it infects. An existing HIV treatment, LASER ART (which stands for long-acting slow effective release antiretroviral therapy), is very effective at stopping the production and insertion of new copies of the virus, but it can't remove genetic code that has already been created. In other words, modern therapies can stop the HIV infection from spreading, but can’t eliminate it entirely. That's why HIV is considered a chronic disease that requires lifelong treatment.
A permanent cure for HIV (and other retroviral diseases) would require removing or inactivating the viral DNA that is mixed with the host’s own genome. Conveniently, cutting through unwanted DNA sequences is CRISPR’s specialty.
In bacteria infected by viruses in natural conditions, special enzymes recognize the intrusion of foreign DNA. They use that DNA as a template to produce guide RNAs, which Cas9, a DNA-cutting enzyme, can use to find its intended targets. The next time a bacterium encounters this foreign DNA, Cas9 can recognize and destroy it. This protects the bacterium from repeated infection by the same viruses.
With CRISPR, scientists can use artificially-created guide RNAs to direct Cas9 to the targets they're interested in. In this particular HIV study, after suppressing the HIV infection with LASER ART, the researchers treated the mice with edited guide RNAs that excised the viral DNA from the genome — actually "curing" the mice of the chronic disease.
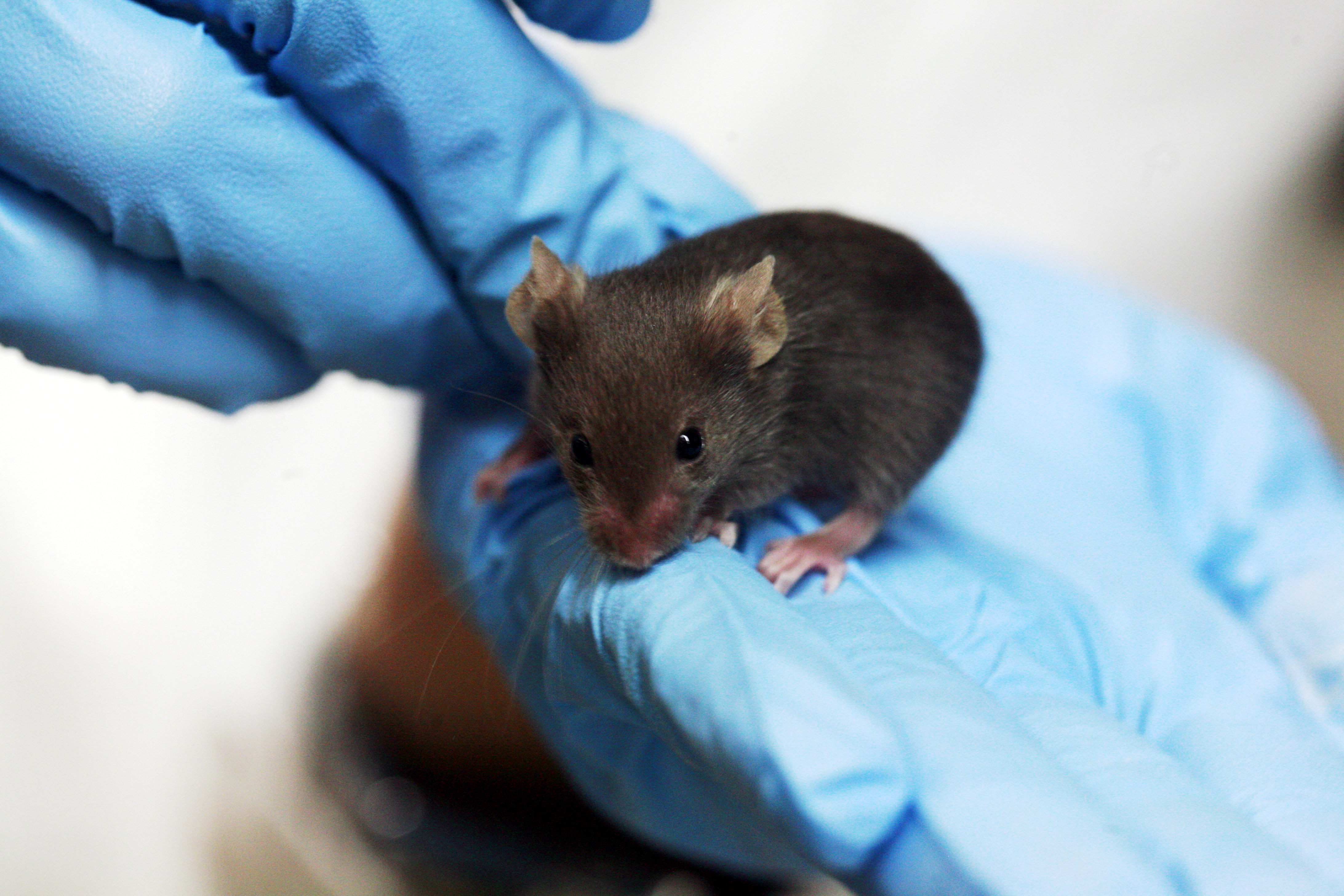
Although this approach is incredibly promising, an important caveat is that the editing worked in less than half the mice.
Rama on Wikimedia Commons
Although this approach is incredibly promising, an important caveat is that the editing worked in less than half the mice. The excision of the viral DNA was incomplete in the rest. It is plausible that the excision efficiency could be improved by increasing the duration of CRISPR-Cas9 treatment. But a worrying possibility is that the HIV virus will quickly develop mutations that help it avoid detection by the synthetic guide RNA. This exact scenario was encountered in a recent attempt to use a similar CRISPR technique to protect the food crop cassava from gemini viruses (note: the lead author of this cassava study, Devang Mehta, is a Massive contributor). Native bacterial CRISPR systems would naturally develop new guide RNAs to combat the new viral strains. But for use in mice, cassava, or humans, scientists might find themselves forced to constantly re-engineer synthetic guides, or to use multiple guides for simultaneous targeting of different parts of the viral genes.
We've seen similar arms races with other types of medical treatments derived from natural sources. The first modern antibiotic, penicillin, was isolated from a mold. Many clinically-useful antibacterial agents have since been isolated from microbes — but the problem of antibacterial resistance is growing. When conventional antibiotics fail, doctors have recently started turning to viruses that can be used to specifically target bacteria to fight off infection, a strategy known as phage therapy.
This demonstration of CRISPR as an antiviral therapy may be among the first of many — but it is part of a longstanding trend of borrowing tools from simple organisms for our own battles.
Peer Commentary
Feedback and follow-up from other members of our community
Bhavya Singh
Microbiology
McMaster University
This is very interesting! Firstly, it is very refreshing to see an article about the ecological role (competition) of CRISPR in the environment, and the potential of using CRISP for antiviral therapies. Also, you bring up a very important point that bacteria could constantly mutate and adapt to combat the antiviral therapy.
I actually had a few questions - do you know why the excision efficiency was only about 50%? Furthermore, this may be a naive question, but how many HIV strains are out there and how different are they from each other? If this were to be used as a therapy for HIV, would the engineered synthetic guides have to be different for each strain?
Thank you for the comments, @bhavya! The actual excision efficiency was higher than 50% of HIV gene copies (to be exact, it was between 60-80% complete excision, and on top of that some undetermined fraction was deactivated by local mutations without being completely cut out of the genome) but in order for a mouse to be free of HIV, every single latent copy in every one of its infected cells must be eliminated or incapacitated. I suppose it would be more accurate to say that in half the mice, the excision efficiency was less than 100%, but anything less than complete elimination means there’s a chance the HIV could come back!
My understanding is that there are two main strains of HIV (this study used HIV-1), and each has many many genetically distinct subtypes. I’m actually not sure if there are targetable sequences common to every subtype, that is an excellent question! But I think a combination approach would be the smartest approach anyway, given HIV’s high mutation rate.